What is a VNA & How to use a network analyzer?
A Vector Network Analyzer (VNA) is like a super important tool for testing things that use wireless technology. It helps make sure that all the cool wireless stuff we use today works properly. Think about it like this: when companies are designing new wireless gadgets, they use simulations on computers to see if their ideas will work. But to make sure those simulations are right, they need to test them with real devices. That’s where VNAs come in. They’re used to check if the real devices match up with what the simulations say.
And when these gadgets get made in factories, VNAs are used again to check that they meet the right standards before they’re sent out to customers. Now, let’s dive into why VNAs are so special compared to other testing gadgets. Imagine you have a magic tool that can both send out signals to test things and also listen carefully to how those things respond. That’s basically what a VNA does. It sends out a known signal to the thing being tested (like a wireless device), and then listens to how it reacts. This helps it figure out exactly how well the device is working.
But there’s more to it than just sending out signals and listening. VNAs are really good at spotting tiny details that other testing gadgets might miss. They can pick up on things like how much signal gets reflected back and how much gets through, which is super important for making sure everything works smoothly.
Now, let’s talk about how VNAs actually do their job After all that, VNAs can measure all sorts of things, like how signals travel through wires or how well antennas work. They can even look at things in different ways, like checking them over time or seeing how they react to different levels of power.
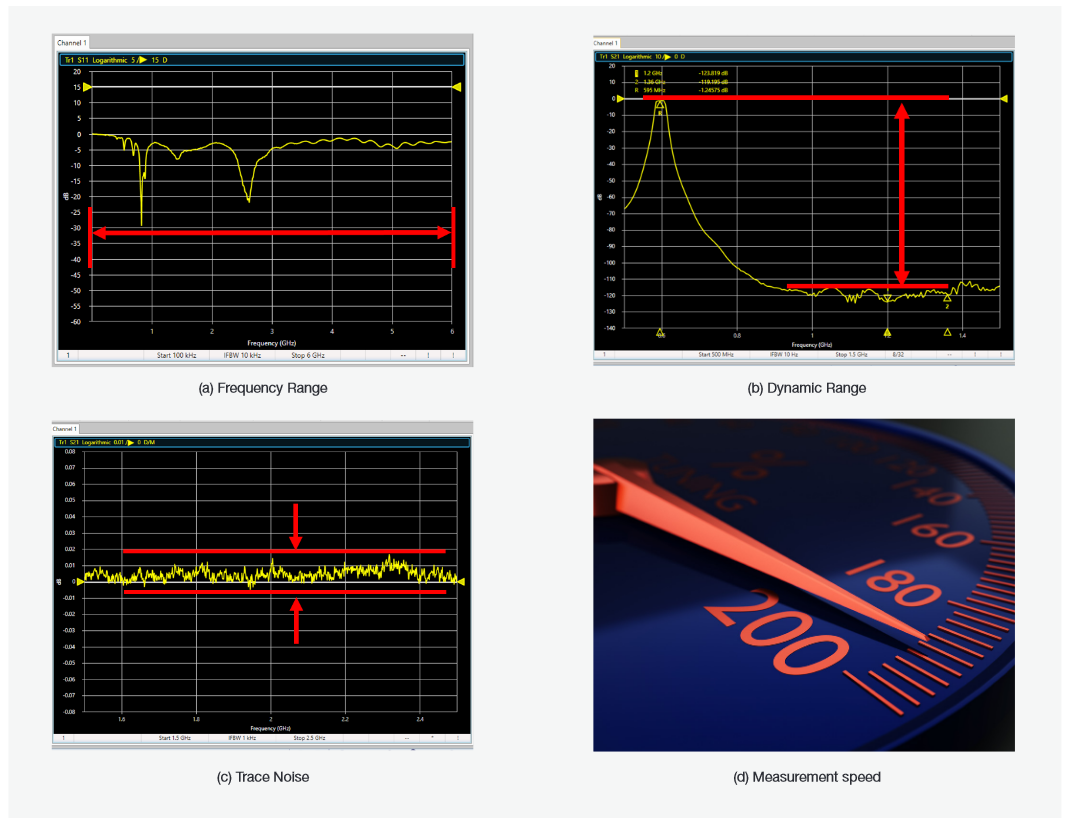
BASIC VNA OPERATION
One unique feature of a VNA is that it contains both a source, used to generate a known stimulus signal, and a set of receivers, used to determine changes to this stimulus caused by the device-under-test or DUT. Figure 5 highlights the basic operation of a VNA. For the sake of simplicity, it shows the source coming from Port 1, but most VNAs today are multipath instruments and can provide the stimulus signal to either port.
The stimulus signal is injected into the DUT and the VNA measures both the signal that’s reflected from the input side, as well as the signal that passes through to the output side of the DUT. The VNA receivers measure the resulting signals and compare them to the known stimulus signal. The measured results are then processed by either an internal or external PC and sent to a display.
There are a variety of different VNAs available on the market, each with a different number of ports and paths for which the stimulus signal flows. In the case of a 1-port VNA, the DUT is connected to the input side of Figure 5 and only the reflected signals can be measured. For a 2-port 1-path VNA, both the reflected and transmitted signal (S11 and S21) can be measured, however, the DUT must be physically reversed to measure the reverse parameters (S22 and S12). As regards to a 2-port 2-path VNA, the DUT can be connected to either port in either direction because the instrument has the capability of reversing the signal flow so that the reflections at both ports (S11 and S22), as well as the forward and reverse transmissions (S21 and S12), can be measured.
KEY SPECIFICATIONS
When determining your needs for a VNA, there are several key specifications to consider. While there are many VNA specifications, there are four top-level specs that can be used to guide your selection process – frequency range, dynamic range, trace noise, and measurement speed.
Frequency range is the first and most critical specification to consider (Figure 3a). For this, it is often good to consider not only your immediate needs but also potential future needs. In addition, while all DUTs have a given operational frequency, for some DUTs you may need to consider harmonic frequencies as well. Active components, such as amplifiers, converters and mixers may need to be tested at their harmonic frequencies which are 2 to 5 times operational frequency. Filters and duplexers may also need to be tested at harmonics of their passband. Although a higher frequency range may be desired, maximum frequency range can be a major cost driver for VNAs.
Dynamic range is the measurable attenuation range from max to min for a specified frequency range (Figure 3b). Based on the desired performance of your DUT, you need to make sure that the magnitude of your maximum DUT attenuation specifications is at least three to six dB less than the VNA dynamic range specification. Most VNAs today offer a very good dynamic range (~ 120 dB) which is sufficient for many applications. Some very high-performance components may require more expensive VNA solutions.
Trace noise measures how much random noise is generated by the VNA and passes into the measurement. It is typically measured in milli-dB (0.001 dB). Trace noise can be a key factor in determining the accuracy of certain components (Figure 3c). An example may be the acceptable level of ripple in the passband of a filter. If you need a certain level of performance to determine accuracy of a signal through a filter, the added VNA trace noise contribution may be a factor.
Finally, one of the other specifications to consider is measurement speed (Figure 3d). Measurement speed is the time it takes to perform a single sweep or measurement. This can be the most critical requirement for high volume manufacturing applications. If you consider a component that is used in a smartphone, there may be billions of components made each year. Reducing the test time at very high volumes is critical to the success of that component. However, for many R&D and low-volume production applications, the VNA measurement speed is not an issue.
Understanding S-Parameters
Since it is generally difficult to measure current or voltage at high frequencies, scattering parameters or S-parameters are measured instead. They are used to characterize the electrical properties or performance of an RF component or network of components and are related to familiar measurements such as gain, loss, and reflection coefficient. To understand how to use a VNA to characterize a DUT, it’s important to understand the basics of S-parameters. Figure 4 walks through a simple process of explaining S-parameters.
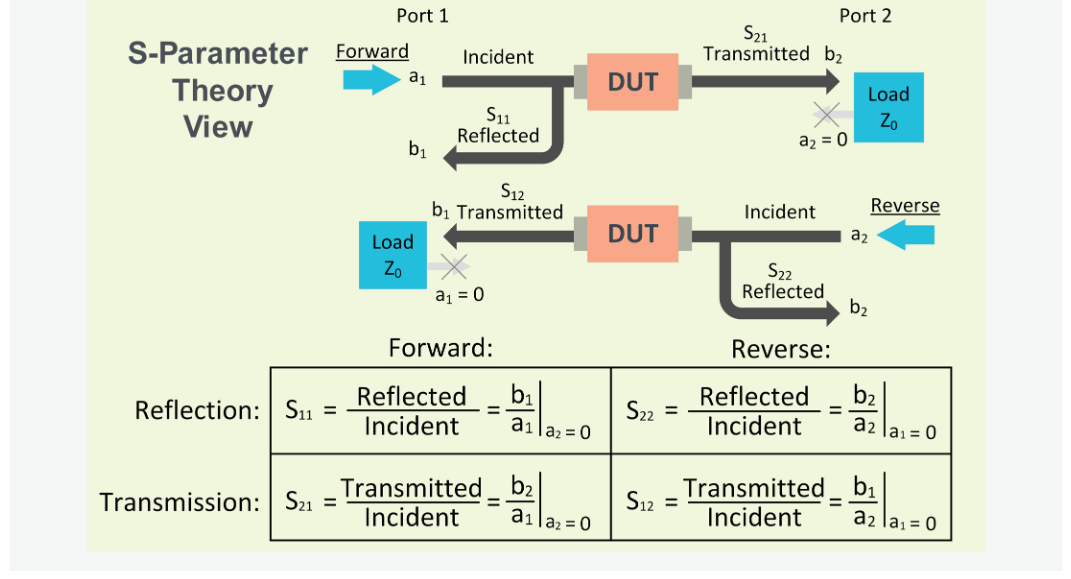
If we start with the Outside View, a VNA typically has two or more ports that simply connect to the DUT – either directly or with the use of cables and adaptors. These ports are labeled, in this case, Port 1 and Port 2.
Next, let’s consider the Inside View. The common practice used to evaluate the behavior of a multi-port network is to use incident waves as excitations at each port and to measure the resulting exiting waves that are either reflected from the port where power is applied or transmitted through the device to the remaining ports. Generally speaking, the waves entering a network or DUT are called incident waves, and the waves exiting a network or DUT are called reflected waves, although each may be composed of a combination of reflections and transmissions from other ports.
The incident waves are designated as an and the reflected waves are designated as bn where n is the port number. Both a and b waves are phasors, having both magnitude and phase at the specified terminals of the network port.
Behind each of the two VNA port connectors is a directional coupler (green boxes in Figure 7). These directional couplers pass the known stimulus signal into either side of the DUT (either a1 or a2
First, a portion of the stimulus signal is taken as a reference signal. S-parameters are defined as ratios of signals coming from various ports relative to this reference. At the same time, some of the stimulus signal is reflected as it enters the DUT (b1 ). The portion of the input signal that is reflected is measured with a receiver connected to Port 1 inside the VNA. The portion of the input signal that enters the DUT generally experiences changes in magnitude and phase as it passes through. The portion that is emitted from port 2 is measured by the VNA receiver on Port 2 (b2 ).
It’s important to note that since the VNA is a bidirectional instrument, Port 2 could also be where the known stimulus is emitted (in that case a2 ), and the measurement process is the same going in the reverse direction.
So now that we know more about how a VNA operates, let’s translate the Inside View into the S-parameter Theory View. By using a (incident) and b (reflective) waves a linear network or DUT can be characterized by a set of equations describing the reflected waves from each port in terms of the incident waves at all of the ports. The constants that characterize the network under these conditions are called S-parameters.
In the Forward case, depicted in Figure 7, Port 1 is transmitting the a1 signal and a matched load is applied to Port 2, resulting in zero signal reflection at the load (a2 = 0). S11 corresponds to the reflection coefficient at Port 1, or ratio of b1 over a1 . S21 is the forward transmission coefficient through the DUT and is the ratio of b2 over a1 .
In the Reverse case, Port 2 is transmitting the a2 signal and a matched load is applied to Port 1 (a1 = 0). S22 corresponds to the reflection coefficient at Port 2, or ratio of b2 over a2 . S12 is the reverse transmission coefficient through the DUT and is the ratio of b1 over a2 .
Note that in the S-parameter nomenclature, Syx, the second number (x) represents the originating port, while the first number is the destination port (y). Theoretically speaking, S-parameter theory can be applied to networks with an infinite number of ports. For example, a 4-port VNA would have 16 S-parameters: from S11, S12, S13, S14, S21 …. S44. These S-parameters follow the same theory and are ratio measurements between each of the specified ports.
Calibration Techniques
Basically, it ensures that the output signal meets the specs and that input signals will be represented accurately. This factory calibration is similar to the factory calibration performed on a spectrum analyzer with a tracking generator.
Having a known stimulus and receivers built within the same instrument gives the VNA a unique capability to perform an additional “user calibration”. As previously discussed, the VNA measures both magnitude and phase, which means that the user calibration performs a vector error correction. This is what makes the VNA one of the most accurate RF test instruments available. User calibration enables the VNA to factor out the effects of cables, adaptors, and most things used in the connection of the DUT. By removing the influence of the accessories, the user calibration allows for the exact measurement of the DUT performance alone. This enables designers to better understand DUT performance when it is placed into a subsystem.
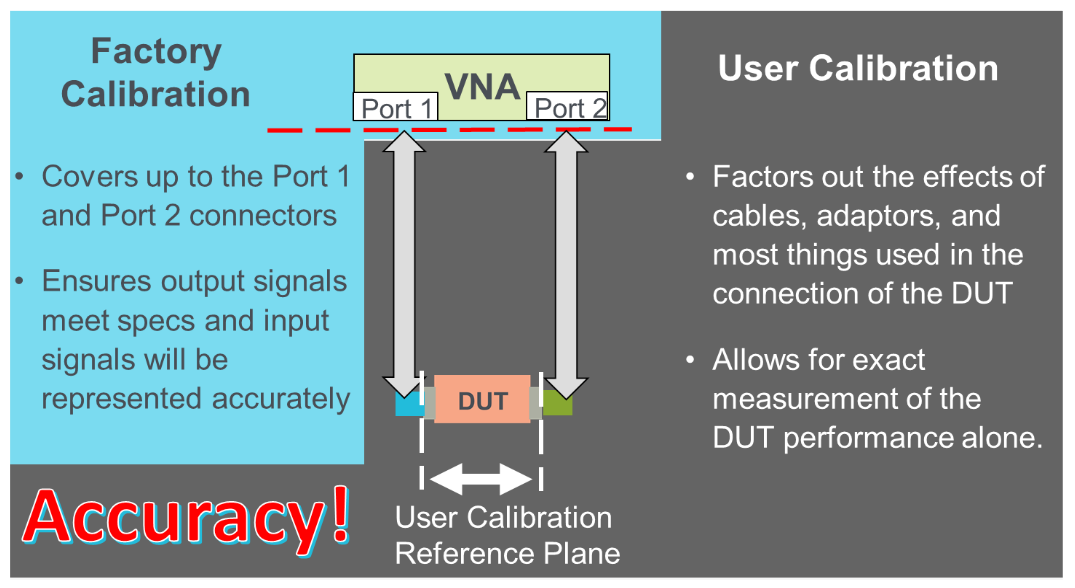
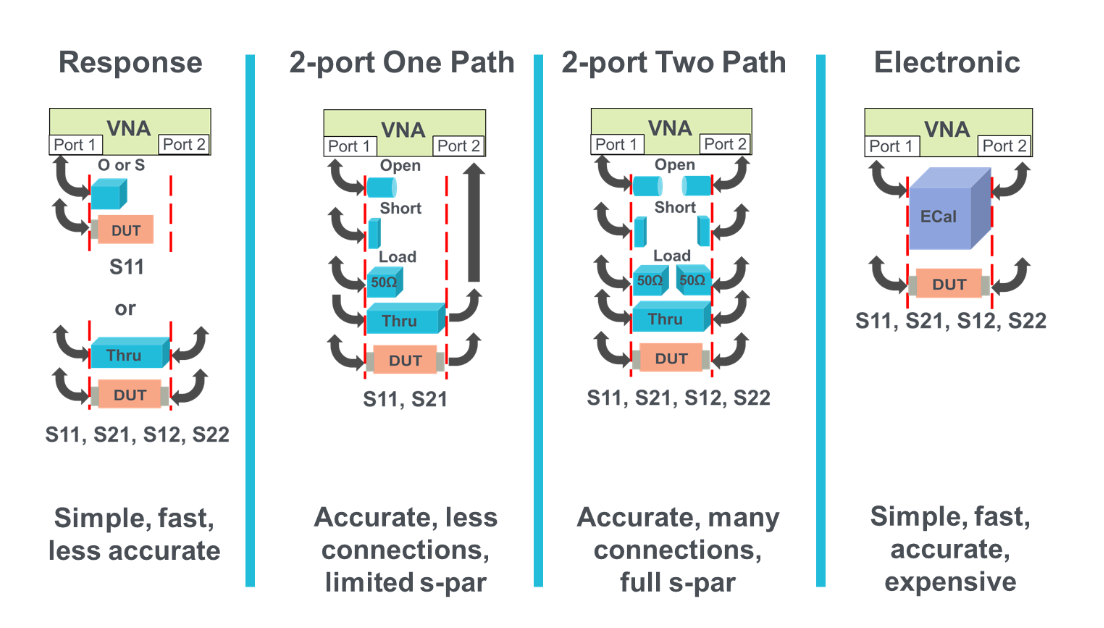
VNA CALIBRATION METHODS
Now that we understand the importance of the “user calibration” in factoring out measurement error, we can go ahead and discuss the different user calibration methods available. There are many different methods of VNA calibration and the complexity that you need is dependent upon your required accuracy and perhaps even your budget (Figure 7). In this section, we review some of the more common methods.
The simplest method is a response calibration. It is fast and easy, but less accurate than other methods. For example, if you only require an S11 or reflection measurement, you may use either an open or a short to measure the test setup response. If only an S21 or transmission measurement is needed, you could use only a thru standard. The response cal is easy to perform and, depending on the accuracy you need, may be sufficient.
Next, there’s the 2-port one path method which is more accurate, but has fewer connections than a full 2-port two path calibration. This method works well when you’re interested in a limited set of S-parameters (e.g. S11, S21, a2 =0). In this case, the VNA will only transmit from Port 1. The benefit is fewer connections during calibration.
The 2-port two path calibration method is essentially the same as the 2-port one path calibration, but with the addition of the open short load measurement on the Port 2 side. This method provides an accurate, full S-parameter measurement capability. The downside is that it requires many connections to be made. The additional steps can lead to potential process errors as you need to measure and replace standards multiple times.
Finally, there is the electronic calibration method. Simply connect the electronic calibration standard and the VNA performs a simple, fast, and very accurate calibration for S11, S21, S12 and S22 – all with a single set of connections. This single connection is valuable as it reduces the likelihood of inserting the wrong standard during the calibration process. Typically, an electronic calibration standard is the most expensive calibration method available. However, they add tremendous value by greatly simplifying the calibration process, while providing highly accurate results.
CALIBRATION STANDARDS
There are several types of VNA calibration standards used in the user calibration depending on the type of calibration method. The most common calibration standard set is referred to as Short, Open, Load, and Thru (SOLT). A VNA user calibration is performed using these known standards with a short circuit, open circuit, a precision load (usually 50 ohms) and a thru connection. It is best if the calibration standard has the same connector type and gender as the DUT. This allows for the DUT or calibration standard to be the only change between calibration and measurement.
Unfortunately, it is not possible to make a perfect calibration standard. A short circuit will always have some inductance; an open circuit will always have some fringing capacitance. The VNA stores data about a particular calibration kit and automatically corrects for these imperfections. The definitions of the standards for a particular calibration kit are dependent on the frequency range of the VNA. In some calibration kits, the data on the male connector is different from the female connectors, so the user may need to specify the sex of the connector within the user interface of the VNA prior to calibrating.
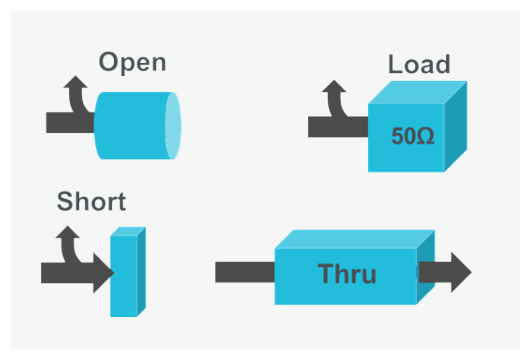
The calibration standards can be physically realized in several different ways (Figure 12). Individual mechanical standards were introduced first, with each standard individually manufactured and characterized. Individual standards offer excellent accuracy and offer flexibility for a variety of test setups.
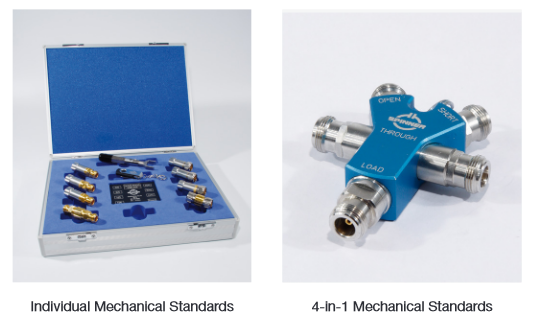
Today, 4-in-1 mechanical calibration kits are available with the open short load and thru integrated into a single mechanical device. As explained earlier, there are also automated electronic calibration standards which are driven by both a computer and a USB. These provide an automatic calibration that is very accurate and less prone to human error by reducing calibration to a single set of connections.
Typical VNA Measurements
VNA’s perform two types of measurements – transmission and reflection (Figure 13). Transmission measurements pass the VNA’s stimulus signal through the DUT, which is then measured by the VNA receivers on the other side. The most common transmission S-parameter measurements are S21 and S12 (Sxy for greater than 2-ports). Swept power measurements are a form of transmission measurement. Some other examples of transmission measurements include gain, insertion loss/ phase, electrical length/delay and group delay. Comparatively, reflection measurements measure the part of the VNA stimulus signal that is incident upon the DUT, but does not pass through it. Instead, the reflection measurement measures the signal that travels back towards the source due to reflections. The most common reflection S-parameter measurements are S11 and S22 (Sxx for greater than 2-ports).
Swept frequency measurements may also measure reflections of the stimulus signal that are incident on the DUT, but are reflected as opposed to being transmitted through the DUT. These S11 (or Sxx) measurements allow the user to check and compare the performance of the DUT to its specification. Example DUTs include antennas, filters, and duplexers. Figure 15 shows an example of an antenna return loss measurement. Note that in the antenna passband, most of the signal is being transmitted so a visible null occurs in the reflection measurement result.
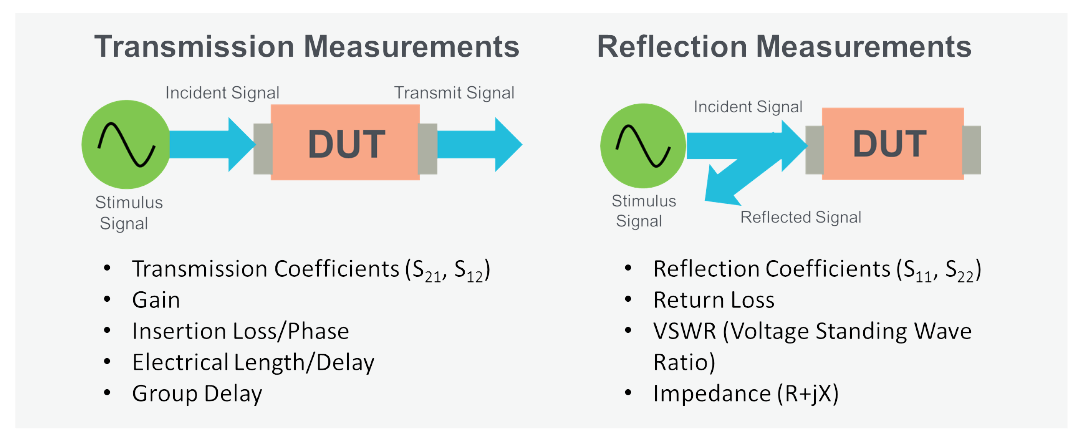
TIME DOMAIN MEASUREMENTS
Some VNAs are capable of using inverse Fourier transforms to convert swept frequency measurements into the time domain. In this way, data displayed in the time domain allows the VNA to be used to find problems in cables and connections by detecting the locations of impedance mismatches or discontinuities as the signal passes through the DUT.
For time domain measurements, the ability to resolve two signals is inversely proportional to the measured frequency span. Therefore, the wider the frequency span, the greater the ability the VNA has to distinguish between closely spaced discontinuities. The maximum frequency span is set by the user and may be defined by either the frequency range of the VNA or the viable bandwidth of the DUT.
The data collected in the frequency domain is not continuous, but a finite number of discrete frequency points. This causes the time domain data to repeat after the inverse of the frequency sample interval. This phenomenon is called aliasing. It is important to set the frequency sample interval correctly to measure the required distance accurately to evaluate the DUT’s performance before aliasing occurs.
Figure 16 shows a VNA measurement of a cable with several adapters. This could be a base station cable running from the base station subsystem to its antenna. The time domain measurement locates the physical distance to the different adapters or potential discontinuities in the cable, which helps locate problem areas or faults.
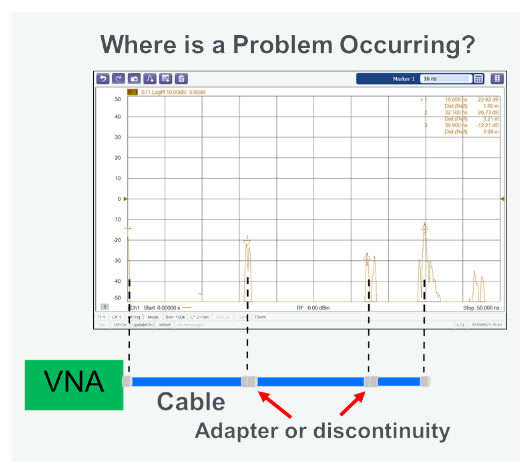
SWEPT POWER MEASUREMENTS
Instead of sweeping frequencies, VNAs may also sweep the stimulus signal’s output power level. For these measurements, the frequency is held constant while the output power is incrementally stepped across a defined power range. This is a common measurement for amplifiers, starting at a low power level and incrementing the power at fractional dB steps.
In the linear region of an amplifier, as the input power increases, the output power increases proportionately. The point when the amplifier output deviates from the linear expectation by 1dB is referred to as the 1 dB compression point (Figure 17). When the amplifier reaches its compression point, it is no longer able to increase its output power as before. For applications that require linear performance of an amplifier, this measurement helps define that specification.
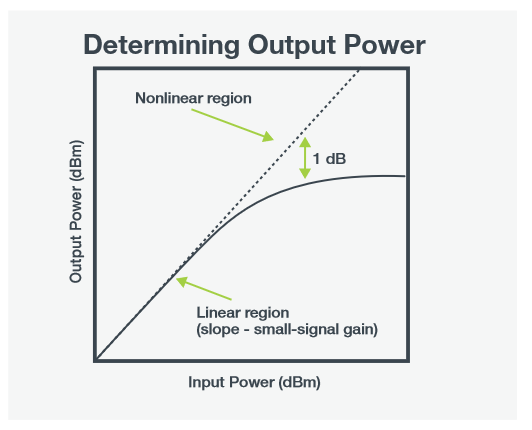
When there’s a need to measure the interaction between multiple ports, you may need a multiport VNA. A true multiport measurement would measure N2 S-parameters and require a VNA with N-ports, where N equals the number of DUT ports. Instead of only S11, S21, S12, and S22, the S-parameters would also include S41 or S43 or S10 11, for example. The true multiport VNA can provide a stimulus signal to each of the ports. Multiport error correction removes systematic errors for the measurement, but requires a complex calibration process where calibration standards must be connected to all possible combinations of ports.
Summary
Now, it is easy to understand why VNAs have helped to make many modern technologies possible. By providing a known stimulus signal to the device under test or DUT, and multiple receivers to measure the response, the VNA forms a closed loop, allowing it to measure the electrical magnitude and phase response of components very accurately. Due to its unique user calibration, the VNA is one of the most accurate RF test instruments available. It allows for careful isolation of the DUT performance by reducing the influence of cables, adapters, and other testing aides.
VNAs test component specifications and verify design simulations. With this accurate level of characterization, engineers can study a circuit or system-level design and rest assured knowing – from the design phase to manufacturing phase – it’s going to function as expected.
thanks Mr. Divyanshu for uploading this content….it’s very useful for me.
Thank You Sir !
I’ve been visiting this site for years, and it never fails to impress me with its fresh perspectives and wealth of knowledge. The attention to detail and commitment to quality is evident. This is a true asset for anyone seeking to learn and grow.
Thanks zencortex!